Balancing act
One day science will restore balance to the genomes of 8p heroes. It will be a long, arduous journey. The first step toward a curative chromosome therapy can be summed up as: aneuploidy, heal thyself.
In collaboration with
Since the dawn of life three billion years ago up to this very moment, chromosomes perform a timeless microscopic Cirque du Soleil inside the nucleus of every mother cell that is poised to divide into two daughter cells. This improbable performance has repeated trillions upon trillions of times across the tree of life over our collective evolutionary history. Critically, the genetic material in a mother cell — in the case of humans, 23 pairs of chromosomes — must be evenly bequeathed to her two daughter cells. Otherwise, the genome is out of balance and the consequences can be catastrophic.
For example, most miscarriages are caused by aneuploidy affecting one or more chromosomes. Just this week, a study of 948 individuals showed that chromosomes are constantly lost or gained or rearranged in tissues across the body, becoming the seeds of cancer that try to sneak past the immune system. Chromosome acrobats, flying near flawlessly every night without a net sometimes fall. There are cases where aneuploidy is not lethal in utero and allows for development of a fetus to term. The most well known case is trisomy 21 (T21), or Down Syndrome.
Trisomy is compatible with life in the cases of chromosome 18 (T18), Edward Syndrome, and chromosome 13 (T13), or Patau Syndrome. Not surprisingly, trisomy of a smaller chromosome that contains less genetic cargo is more likely to be tolerated than trisomy of a larger chromosome that contains more genetic cargo. Stem cells in particular may be particularly sensitive to aneuploidy because they need to function nearly flawlessly throughout our lives.
To wit, multi-month in vitro passaging of trisomic induced pluripotent stem cells (iPSCs) from patients with Down Syndrome, Edward Syndrome, and Patau Syndrome leads to the spontaneous generation of disomic cells that lose their toxic third chromosome and regain disomy, which confers a selective growth advantage over the trisomic founder cells. A study from 2019 on Down Syndrome iPSCs is dispositive:
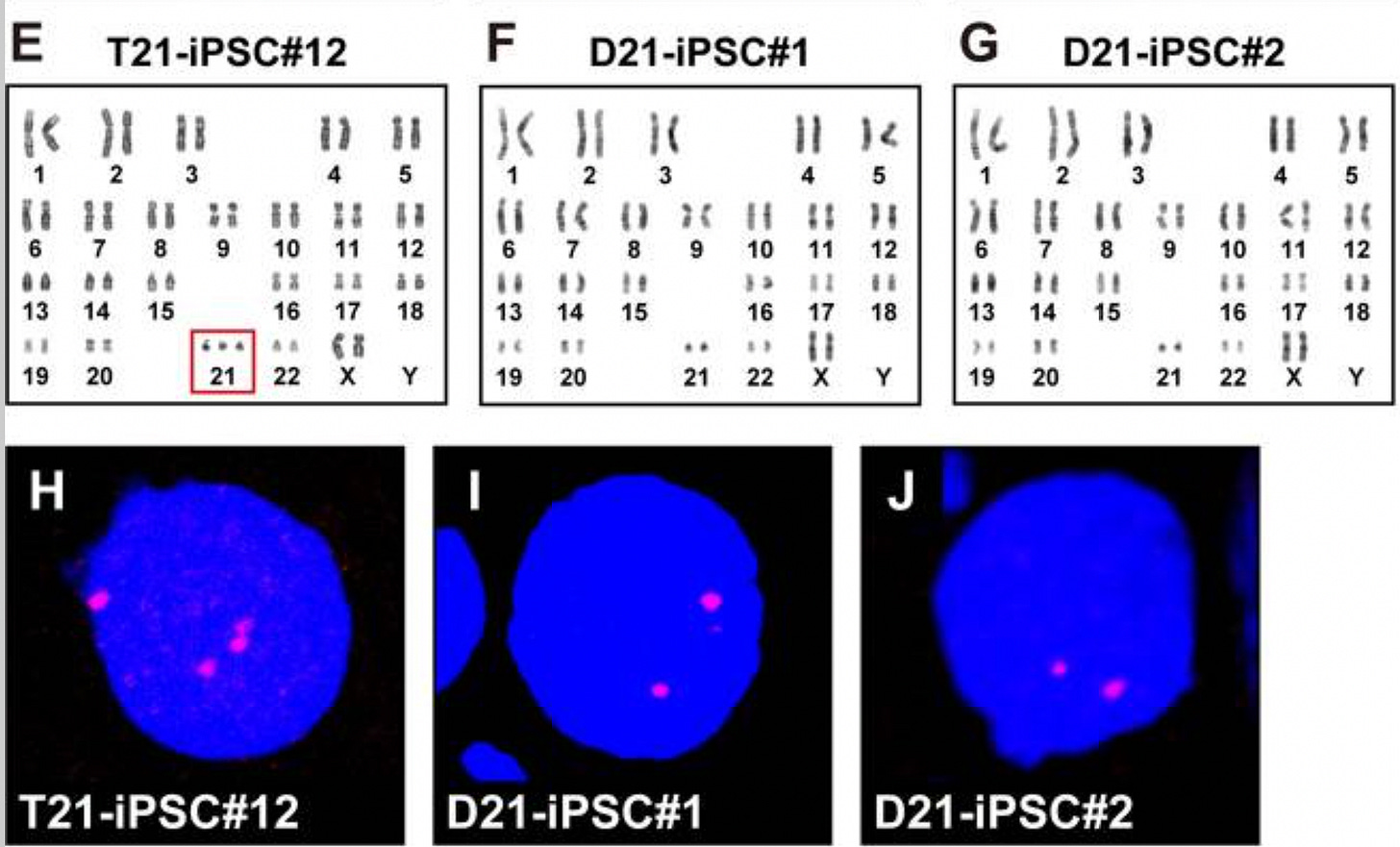
What about 8p, where the duplicated segment can be as large as 30 megabases, which is the same size as the entire length chromosome 21? For inv/dup/del 8p heroes, it’s not just a duplication it’s also a 1-10 megabase deletion, and an inversion just for kicks. Is the rearranged 8p chromosome toxic to cells like the extra copies of chromosome 13, 18 or 21? Though keep in mind that in T21, T13, and T18, the extra genetic material is a whole freely segregating chromosomes, whereas in 8p it is not.
As stated in the Cure Roadmap published a year ago, Project 8p set out to answer that question and others in collaboration with Professor Jason Sheltzer’s lab at Yale University. This project is actually a twofer in the sense that it yields not only discoveries but also practical deliverables. Not only will we be able to assess the intrinsic cytotoxicity of the rearranged 8p chromosome in iPSCs, but in the process we will be able to isolate disomic but otherwise genetically identical (isogenic) 8p IPSC clones. In the business we call that an isogenic corrected control line, which itself is a non-negotiable asset for downstream research applications like chromosome therapy.
Everyone at this point in time has probably heard of gene therapy: replacing a single broken gene with an intact working copy. When genetic lightning strikes a chromosome, imagine gene therapy but on anabolic steroids: replacing or fixing dozens if not hundreds of storm-tossed genes at once.
8p rearrangements are a complex coalition of copy number variant disorders. One cohort of 8p heroes has lost one copy of the genes located in deleted 8p segments, resulting in haploinsufficiency. Another cohort of 8p heroes has an additional copy of each gene — meaning three copies instead of the usual two — in 8p duplicated segments. The largest cohort of 8p heroes has both duplications and deletions and the aforementioned inverted segment.
Confusingly, the body sometimes doesn’t mind if some genes are present in half or double the normal amount, but cares deeply when the genome as a whole is out of balance. At the same time, some genes have the dubious honor of being “driver genes,” as appears to be the case with the gene called RELN aka Reelin. That means the body cares a lot whether a specific gene is in balance, i.e., present in two copies, one from mom and one from dad.
Building on their success in studying aneuploidy in the cancer space, Sophia Lee in the Sheltzer lab at Yale University applied those learnings to 8p. As a first step, the 8p pioneer induced pluripotent stem cell (iPSC) trio — mom, dad and 8p hero — was karyotyped. That’s the technical term for assessing how many copies of each chromosome are present in a sample from a patient. Normally, there are two copies of each of the 22 autosomal chromosomes. The 23rd pair of sex chromosomes — XX in girls, XY in boys — can also be detected.
Zooming in on the affected proband, which is genetics-speak for the affected child, we can clearly discern both the deleted (haploinsufficient) 8p segment at the half of the normal copy number as well as the duplicated (trisomic) 8p segment at double the normal copy number. The Y chromosome is undetectable, as expected since the proband is female. Crucially, besides 8p there are no other detectable chromosomal copy number variants (CNV) detected. That means the results won’t be compromised by an unwanted CNV outside of the 8p region.
Next, Sophia and Jason devised a devilishly simple quantitative PCR (qPCR) assay for tracking changes in chromosome 8 copy number in cells passaged in the lab over many weeks. It works by quantifying the amount of a marker gene as a proxy for the copy number of an affected segment of 8p. The CSMD1 gene resides in the proband’s deleted 8p segment, while the MICU3 gene is located in the proband’s duplicated 8p segment. As expected, only a single copy of CSMD1 is detected in the proband in contrast to the two copies detected in the parents and an unrelated healthy control line. Correspondingly, one extra copy of MICU3 is detected in the proband.
The next phase of the experiment is a waiting game. As cells divide in culture, there’s selection pressure to grow faster and faster. If the damaged 8p chromosome is intrinsically toxic, the selective pressure to jettison the damaged chromosome while at the same time making an entire extra copy of the unaffected chromosome 8 is enormous.
After more than 100 days of passaging in the lab, Sophia assessed the copy-number status of chromosome 8 using the CSMD1 deleted-segment marker and the MICU3 duplicated-segment marker. The results are fairly definitive: there was no spontaneous return to genomic balance. Therefore, we can likely conclude that the damaged 8p chromosome is not inherently cytotoxic — at least in undifferentiated iPSCs — like the three well-known trisomies. As a community we still have to digest this result and its implications, but for the purposes of generating a euploid 8p proband clone, just letting 8p cells divide over time wasn’t sufficient to do the trick.
In his original proposal, Jason mapped out several alternate selection strategies in the event that the spontaneous path didn’t pan out. The first trick up their sleeve was to treat proband cells with a compound called AZ3146, which inhibits the mitotic kinase MPS1. When that happens, the probability of what are called missegregation events increases significantly. Missegregation events mean errant cell division resulting in whole-chromosome gains and losses.
Dozens of clones were assessed for chromosome 8 copy number changes, in any direction. What we want to see are clones that have an identical profile to the control, which is diploid (two copies of each gene). Most of the clones did not exhibit any change in copy number; they stayed exactly the same. Clone 37 exhibited a quadrupling of the CSMD1 marker gene, implying that just the deleted 8p segment was present in tetrasomy, or four copies. Interestingly, several clones show an increase in copy number of both CSMD1 and MICU3, not just one or the other.
For example, clones 31 and 33 look promising. CSMD1 is clearly increased in copy number from 1 to 2. MICU3 also appears to be increased in copy number from 3 to 4, though the result is more striking for clone 33. These data suggest that clones 31 and 33 gained an entire copy of chromosome 8, or a trisomy comprised of the original unaffected paternal chromosome 8, a newly duplicated unaffected paternal chromosome, and the damaged maternal chromosome 8.
Whole genome sequencing of clones 31 and 33 confirmed the presence of a third copy of chromosome 8. The yellow arrows show the q (long) arm of chromosome 8 at > 1 copy number. Consistent with the interpretation of a gain of an unaffected full-length chromosome 8, the deleted 8p segment and the duplicated 8p segment also increase in copy number to the same extent.
In the next phase of this foundation-laying project, clones 31 and 33 will be further passaged in the hopes that the rearranged 8p chromosome is spontaneously lost during a cell division. Sheltzer lab has other tools available to target the rearranged 8p chromosome for elimination, e.g., KaryoCREATE.
Once we have proband iPSC clones with restored disomy, the final phase of the project will involve a head-to-head comparison to the original rearranged/damaged 8p chromosome proband iPSC line. Phenotypes that will be examined are doubling time, whole-transcriptome RNAseq profiling, and neural rosette formation. A similar workup was performed in the above-cited 2019 paper describing spontaneous recovery of disomy of Down Syndrome iPSCs in long-term cell culture.
Unfortunately, genomic imbalance is not just a threat to life starting in the embryo. Aneuploidy is a silent assassin that is constantly taking out individual cells in your body all the time, and the rate accelerates as we age faster than our immune system can keep up. Aneuploidy is one of the pillars of aging.
So when anyone asks why they should care about 8p, aneuploidy is why. Project 8p is leading this charge and making the case for why 8p will be an experimental sandbox for developing curative chromosome therapies one day in the future.